2017 Symposium
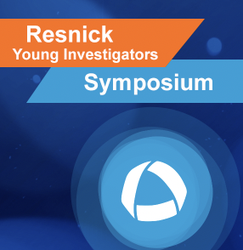
2017 Recap
The Resnick Young Investigators Symposium celebrates innovators in the science and technology of sustainability. The program highlights young researchers whose work shows great promise in tackling key science and engineering challenges in sustainability. The 2017 Resonate Award winner was announced during the program.
2017 Speaker Lineup
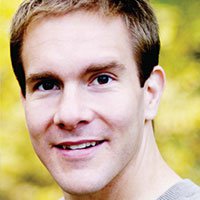
Shane Ardo
Assistant Professor of Chemistry, and Chemical Engineering and Materials Science at UC Irvine
Is Water the Ideal Semiconductor for Direct Solar-Cell-Driven Desalination?
One in ten human beings does not have access to clean potable water, a number that the United Nations predicts will more than quadruple in the next 13 years mostly due to population growth in developing nations. While oceans constitute an enormous supply of water, the concentrated salt present in sea water is poisonous to people. Therefore, there exists an urgent need for rapidly-deployable, reliable, affordable, and efficient technologies to desalinate salt water for human consumption and agriculture.
My group is pioneering a new solar-energy-conversion technology that we think can help supply potable water to those people that need it most. Central to our approach is an innovative mechanism for light-to-ionic energy conversion inspired by traditional inorganic solar cells and photosynthesis in Archaea and that uses water as the semiconductor. We have shown that in one polymeric material the energy in sunlight is directly converted into ionic power, a process that theoretically can desalinate ocean water 20 times more rapidly than state-of-the-art solar thermal distillation can.
Our enabling materials breakthrough utilized covalent photoacid-dye-modified ion-exchange membranes. Absorption of visible light by photoacid molecules decreased their pKa values, which resulted in liberation of protons that were then collected at membranes selective to specific ionic charges. The resulting ionic photocurrent and photovoltage were consistent with a mechanism where ions were pumped against a concentration gradient to produce power. It is our hope that a technology based on our research can help overcome some of the urgent global challenges surrounding clean water scarcity.
About Shane
Shane obtained a BS in mathematics, with a minor in computer programming, from Towson University and subsequently worked as a software engineer, community college instructor, and high school teacher prior to attending graduate school. Shane obtained an MS in nutrition from the University of Maryland, College Park followed by MA and PhD degrees in photo-physical inorganic chemistry from the Johns Hopkins University, where he worked for Prof. Jerry Meyer. He then worked for Prof. Nate Lewis as a DOE-EERE Postdoctoral Research Awardee at the California Institute of Technology until 2013.
Since that time, Shane has been an Assistant Professor at the University of California, Irvine in the Department of Chemistry and holds a joint appointment in the Department of Chemical Engineering and Materials Science. His research interests are driven by the pursuit of understanding and controlling energy conversion mechanisms for applications in solar fuels devices, photovoltaics, solar seawater desalination, fuel cells, and redox flow batteries.
Shane was recently named one of five inaugural Moore Inventor Fellows, is a recipient of a Beall Innovation Award, and was named a Sloan Research Fellow and a Cottrell Scholar. His research group is also supported by funding from the U.S. Department of Energy's Office of Energy Efficiency and Renewable Energy and the U.S. National Science Foundation's Chemical Catalysis Program.
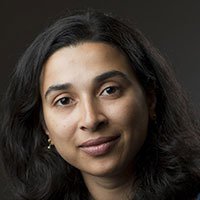
Nandini Ananth
Assistant Professor in the Department of Chemistry and Chemical Biology at Cornell University
Designing Organic Molecules for Solar Photon Harvesting
Organic solar cells offer an environmentally friendly and inexpensive alternative to their inorganic counterparts, but to-date their applications have been limited by relatively low efficiencies. Recently, Singlet Fission (SF) has emerged as a particularly promising route to high-efficiency organic photovoltaics, however the lack of a fundamental understanding of the process and the small number of chromophores that exhibit this property have hindered progress.
In this talk, we present our theoretical investigations into the detailed mechanisms of SF that yield a priori design principles for novel organic chromophores. SF is a phenomenon where a single exciton created by photon absorption spontaneously splits into two long-lived triplet excitons that may undergo independent charge separation at a donor-acceptor interface.
We employ a combination of electronic structure calculations and quantum dynamic simulations to uncover two distinct pathways for SF in non-bonded and covalently bonded pentacene dimers. In each case, we identify the underlying energetics, vibronic couplings, and the intra- and inter-molecular interactions that may be used to tune SF rates. Furthermore, using novel orbital analyses, we uncover correlations between bonding topology and enhanced visible light absorption in acene-based molecules.
Combining the insights from these studies, we generate design principles for next-generation organic chromophores, and working with experimental collaborators we verify them.
About Nandini
Nandini Ananth is an Assistant Professor in the Department of Chemistry and Chemical Biology at Cornell University. She obtained her Bachelors in Chemistry from Stella Maris College, Chennai followed by a Masters from the Indian Institute of Technology at Madras.
Her undergraduate research included implementing logic gates for quantum computing using Nuclear Magnetic Resonance and Semiclassical Dynamics. She obtained her Ph.D. developing Semiclassical methods for quantum dynamic simulations in complex systems working with William Miller at the University of California, Berkeley.
After graduation, she accepted a position as postdoctoral scholar in Thomas Miller's group at Caltech where her research focused on developing path-integral methods for the simulation of electronically nonadiabatic processes in the condensed phase.
Since beginning at Cornell in 2012, her research has focused on developing and using techniques based on semiclassical theory and the path integral formulation of quantum mechanics to simulate charge and energy transfer processes in complex chemical systems. Target applications involve designing novel materials relevant to renewable energy technologies including organic photovoltaics and transition metal complexes for photocatalytic water splitting.
During her time at Cornell she has received several awards including the GPSA Faculty Mentor Award, Cottrell Scholar Award, the Sloan Foundation Research Fellowship, NSF CAREER Award, NSF EAGER Award, and the Army Research Office Young Investigator Award.
The Age of Global Fresh Water Resources
The time that rain and melting snow take to flow into a river or to a location under the ground impacts nutrient and contaminant transport, and is therefore important to sustaining healthy humans and ecosystems. These ‘water ages' of streamflow and groundwaters have remained poorly understood. Here, we use stable and radioactive isotope compositions of rain, snow, groundwater and streamflow to map the relative amounts of younger versus older water (i) flowing in rivers, or (ii) discharging from groundwater wells.
First, our research shows that ~1/3 of global streamflow is recent rain or snow that fell and flowed to the stream in less than ~2.3 months. This young streamflow is detectable in the great majority (~90%) of surveyed streams, implying that most catchments can convey precipitation to the stream channel quite quickly, possibly leaving little time to detect some soluble pollutants before they reach aquatic ecosystems.
Second, we show that most of the fresh water on the planet is ancient water that has been stored underground form more than 10,000 years. While vast, these ‘fossil' groundwaters are also shown to be vulnerable to modern-era pollutants, emphasizing that both water quality and sustainability should be considered when managing these deep water resources.
About Scott
Scott Jasechko is an assistant professor of water resources at the University of Calgary. He completed his masters at the University of Waterloo and his doctorate at the University of New Mexico before joining the University of Calgary in January of 2015.
Scott's research focuses on fresh water resources, and uses large datasets to understand how rain and snow transform into river water and groundwater resources.
Scott is an active member of the American Geophysical Union. His work has been recognized by numerous early career awards, including the Young Scientist Award from the Canadian Geophysical Union (2016) and the Horton Hydrology Research Award from the American Geophysical Union (2013).
In November 2017, Scott shall join the Bren School of Environmental Science & Management at the University of California, Santa Barbara.
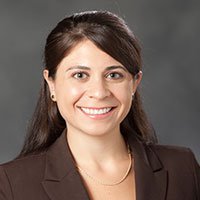
Desirée Plata
Assistant Professor of Chemical & Environmental Engineering; Associate Director for Research, Center for Green Chemistry & Green Engineering at Yale
Can Controlling Matter at the Atomic Scale Solve Global-Scale Problems?: Environmental Optimization Enables Precision Carbon Nanotube Synthesis
Carbon nanotubes (CNTs) are often proposed as the material solutions to environmental challenges facing society (e.g., energy storage and water treatment technologies), but their production by catalytic chemical vapor deposition (CVD) is one the least efficient industrial practices ever developed. Carbon conversion efficiencies range from 0.0001-10% and energy consumption is high compared to other industrial practices (e.g., 107 kJ/kg), even after 15+ years of optimization. Furthermore, atomic-scale control of the CNT nanostructure is not yet possible due to a lack of mechanistic understanding, and this has limited realization of many of the most promising applications of CNTs. Nevertheless, carbon-based nanomaterials remain exceptional with respect to their predicted (and demonstrated) material properties, and discovery of the bond-building steps controlling CNT formation could enable both reduction in the environmental impacts of the process, as well as improved performance of the nanomaterial-enabled devices.
Using in situ CNT height measurements and complimentary gas emissions analysis of volatile organic compounds (VOCs) and polycyclic aromatic hydrocarbons (PAHs), we identified thermally generated compounds that were correlated with CNT formation rate. Direct delivery of these critical precursors (primarily C2-C4 alkynes) in a cold wall reactor to a locally-heated catalyst support enabled greater than 10-fold improvements in carbon conversion efficiency, corresponding order-of-magnitude reductions in emissions, and two-fold energetic savings. Subsequently, we demonstrated that alkynes were responsible for rapid CNT growth and that structural elements of those persisted into the bulk CNTs.
The chemical studies presented here shed new light on the current understanding of CNT synthesis, suggesting that a metal-catalyzed polymerization reaction is responsible for CNT formation. This fundamental contribution is demonstrative of the powerful potential interactions between environmental engineers and materials scientists: where early optimization of environmental, performance, and costs metrics could enable rigorous, sustainable material design to solve global challenges.
About Desirée
Desirée Plata's research seeks to maximize technology's benefit to society while minimizing environmental impacts in industrially important practices through the use of geochemical tools and chemical mechanistic insights, with a particular focus on energy technologies.
Plata earned her Phd in chemical oceanography and environmental chemistry from the Massachusetts Institute of Technology and the Woods Hole Oceanographic Institution's Joint Program in Oceanography (2009) and her bachelors degree in chemistry from Union College in Schenectady, NY (2003).
Plata is an NSF CAREER Awardee (2016), an Odebrecht-Brasken Sustainable Innovation Awardee (2015), a National Academy of Engineers Frontiers of Engineering Fellow (2012), and a two-time National Academy of Sciences Kavli Frontiers of Science Fellow (2011, 2013).
Optoelectronic Properties of Near Single-Crystalline Halide Perovskites
The precise control over stoichiometry, microstructure and crystallinity of nanoscale materials from the atomic to the macroscale using low temperature solution-based approaches has been a fundamental bottleneck that has existed for nearly three decades. When assembled into thin-films using solution-based approaches, the beautiful physics visualized at a single element level are overshadowed even by a small degree of polydispersity resulting in low-efficiency, non-durable and non-reproducible devices.
My research over the past five years has attempted to overcome some of these key challenges by developing unique and innovative low-temperature methods to integrate materials into near single-crystalline thin-films, which has led to high efficiency devices with excellent durability.
In my talk, I will describe these approaches demonstrated on bulk and 2D perovskites materials system, which has lacked a systematic structure-property due to the high degree of variability in composition, crystalline quality, and grain-size with different thin-film processing approaches. This variability has led to multiple and often contradictory interpretations of experimental data.
I will describe our unique thin-film crystal growth approach to grow near single-crystalline perovskite thin-films, which results in thin-films with properties on par with high-quality direct band-gap semiconductors such as gallium arsenide. Importantly, the degree of crystallinity has direct consequences on the overall performance of devices but also dramatically influences their stability.
I anticipate that our results provide the key foundation for the fundamental and technological development of hybrid perovskite-based materials for high-efficiency and stable devices for the next generation clean energy applications.
About Aditya
Aditya Mohite is the PI of the Light to Energy team and directs an energy and optoelectronic devices lab working on understanding and controlling charge and energy transfer processes occurring at interfaces created with organic and inorganic materials for thin-film clean energy technologies.
His research philosophy is applying creative and "out-of-the-box" approaches to solve fundamental scientific bottlenecks and demonstrate technologically relevant performance in devices that is on par or exceeds the current state-of-the-art devices.
He has published more than 90 peer reviewed papers in journals such as Science, Nature, Nature Materials, Nature Nanotechnology, Nano Letters, ACS Nano, Chemical Society Reviews, Applied Physics Letters and Advanced Materials amongst others. He has also delivered more than 60 invited talks.
2017 Program
Opening Comments
Is Water the Ideal Semiconductor for Direct Solar-Cell-Driven Desalination?
- Dr. Shane Ardo, Assistant Professor of Chemistry, and Chemical Engineering and Materials Science at UC Irvine